Actuation on Different Length Scales
The combination of dipolar nanoparticles with functional polymers provides options for a broad range of actuation, controlled by external factors such as temperature, AC or DC magnetic fields, and catalytic reactions.
Magnetic activation of thermoresponsive effects The heat dissipation observed in magnetic nanoparticles under the influence of suitable AC fields can be used for local heat production as a trifgger for thermal effects in soft matrices. Examples originating from our lab include shape memory polymers and liquid-crystalline elastomers that are filled with magnetic nanoparticles that serve as antenna for the AC field and result in a homogenous heat evolution throughout the bulk phase and fast response times.1-4
Similarly, the dispersion properties of thermoresponsive magnetic colloids can be remote-controlled and manipulated by external AC fields.5 Following this principle, the particles can be switched from dispersed to precipitated, and release systems can accordingly be realized.6-10
Magnetic hydrogels We investigate a novel class of ferrohydrogels that possess a direct covalent coupling between the particles and the polymer network segments. Due to a surface functionalization CoFe2O4 spheres and alpha–Fe2O3 ellipsoids act as multifunctional crosslinker. From this it follows that the swelling properties depend directly on the fraction of incorporated particles.
The introduced particle species are magnetically blocked or ferromagnetic so that their orientation in external magnetic fields is accompanied by mechanical particle rotation. Due to the covalent coupling between particles and polymer segments the magnetic force of the particles is transmitted to the polymer. This allows the investigation of the particle dynamics and the counter forces of the polymer matrix concerning viscous and elastic effects by analyzing the magnetic properties in relation to hysteresis effects and the initial susceptibility of the integrated particles.
From the magnetization curves we get information about the particle dynamics and the matrix properties on the length scale of the particles. We have already showed the conclusion of the elastic moduli from the local restoring force via magnetization measurements for the CoFe2O4 linked gels and the results are in accordance with expectations from statistical thermodynamics.2
Nanoswimmers The propulsion mechanisms of bacteria and related swimmers is of inspiration for the design of nanoscopic swimmers with controlled, directed motion. Our concept is the employment of magnetic nanoparticles as the controlling unit that can either directionally or periodically manipulated by external fields. In order to allow for further symmetry break, magnetic snowman-like particles are created that are either catalytically active and can be propelled by chemical fuel, or site-specific functionalization. The challenging task is deal with the overwhelming Brownian motion and the low Reynolds number.
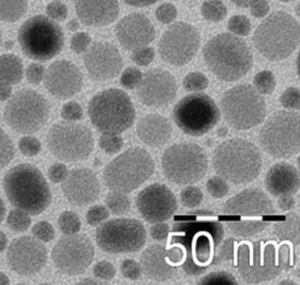
The particle motion can be observed and analyzed by dark field light scattering microscopy. Protocols are developed that allow to extract particle velocity and the differentiation between diffusive and propulsive movement. The translational and rotational motion with and without the influence of magnetic fields is extracted with DLS/DDLS and AC susceptometry.15-16
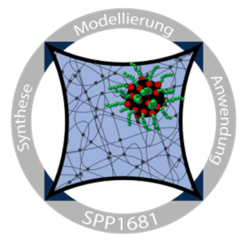